Introduction
IN PART I OF THIS 3-PART series, we outlined the definition of ocular biomechanics and kinematics and described the mechanical laws under which they operate.1 In this article, we describe the specific elements and motions responsible for achieving Dynamic Range of Focus (DRoF), including the phases of vision from accommodation to disaccommodation (near to far) and disaccommodation to accommodation (far to near). The ciliary muscles (CMs) and Bruch’s membrane-choroid complex (BMCC) represent the dynamic engine of DRoF. Interpreting the types of bidirectional forces produced by the critical moving structures during DRoF is essential to understanding the mechanics of the "Kinematic Chain" of events that occur to facilitate the fine-tuned dynamic focusing power our eyes can perform. The aim of this article is to provide a deeper dive into the anatomy and physics of the mechanisms that equip our eyes with DRoF capability and to further explore the elements of this sophisticated mechanism responsible for the adjusting power of the lens.
Three Phases of Dynamic Range of Focus
Dynamic Range of Focus is a term used to describe the dynamic focusing function of the eye consisting of 3 dynamic phases: (1) prestretch-accommodation; (2) accommodation-disaccommodation; and (3) disaccommodation-accommodation. Complex biomechanical interactions occurring between anatomical components, each performing a specific function, are integrated to create the chain reaction, which in turn produces a series of forces that ultimately change the shape and power of the internal optic of the eye—the crystalline lens.
Each phase consists of a distinct posture of the crystalline lens as follows.
- Phase 0: Here, we define prestretch as the maximum point of disaccommodation, where all anatomical structures are taut, and the crystalline lens is in the stretched and flattest posture.
- Phase 1: Accommodation is the phase during which the crystalline lens shape and refractive power change to focus light and obtain a clear retinal image for a range of distances from far to near.
- Phase 2: Disaccommodation is the phase during which the crystalline lens shape and refractive power change to focus light and obtain clear retinal images for a range of distances from near to far.
These mechanisms produce a sophisticated and precise focusing power dynamically across a range of distances in both directions. The focusing function that increases the power of the crystalline lens to see at near is called “accommodation” while the focusing function that decreases which decreases power the power of the crystalline lens to see at far is called “disaccommodation.”2
Action of the Ciliary Muscle Contractions
There are 3 types of contractions that muscles undergo, including isometric, concentric, and eccentric contractions. Isometric contractions are holding contractions that do not undergo a range of movement3 (Figure 1). The functions of the biceps muscle are shown to provide a visual example of the actions of the CM contractions during phases of DRoF.
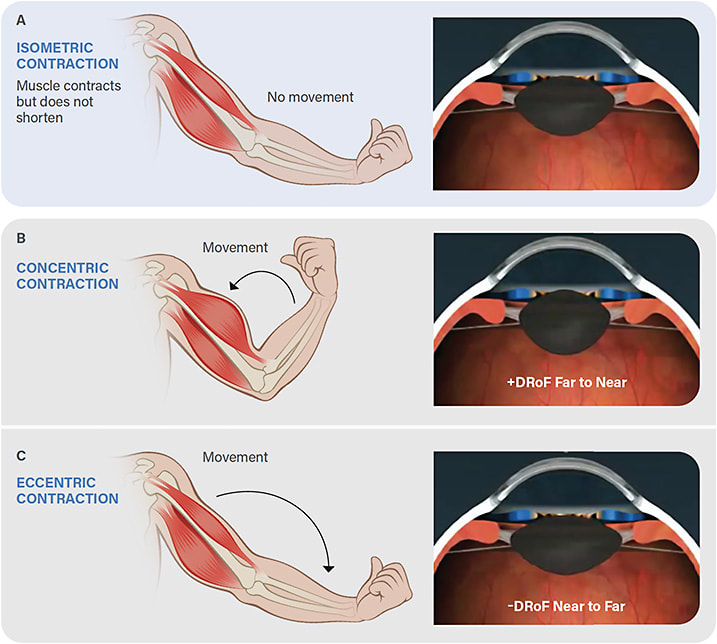
Isometric contraction is a static contraction in which the muscle does not notably change length, and there is very little movement4 (Figure 1a). Reading for a long period of time at a set distance is an example of an isometric contraction in the CM, in which the lens would be focusing in the same position for an extended period of time.
Concentric contractions increase force as the muscle shortens, while the muscle insertion moves toward its origin3 (Figure 1b). For the biceps muscle, this process is called “flexion,” and in the DRoF model, it is referred to as “+DRoF” since the change in focusing power switches from negative to positive. During this phase, the lens shape changes from a flatter biconvex lens shape (disaccommodation) to a rounder biconvex lens shape (accommodation). Like the bicep curl, the CM undergoes a concentric contraction during accommodation. In this phase, the CM undergoes a shortening of the muscle fibers, resulting in the upward pull of the pars plana and relaxation of zonular and capsular tension. This process allows the lens to round up due to its natural elasticity. An example of +DRoF would be switching views while driving from looking at the road ahead to checking the speed limit on the dashboard. Eccentric contractions increase force as the muscle lengthens, while the muscle insertion moves away from its origin.3
The CM undergoes an eccentric contraction during disaccommodation, similar to performing a negative exercise in active lengthening (Figure 1c). For the biceps muscle, this process is called “extension,” while in the DRoF model, it is referred to as “-DRoF” since the change in focusing power switches from positive to negative. During this phase, the lens shape changes from a rounder biconvex lens shape (accommodation) to a flatter biconvex lens shape (disaccommodation). An example of -DRoF would be switching views from reading one’s mobile phone to viewing a program on the television.
Key Functional Anatomy of DRoF
By intelligent design, the eye’s anatomy correlates with its specific function. It is essential to define the roles of the structures that conduct the specific functions of DRoF to understand the complex biomechanical interactions that comprise the focusing mechanisms in our eyes. Below is a list of the key functions that allow the fine-tuning of DRoF to be possible.
Ciliaris musculi (ciliary muscles): The CM has 3 fiber directions, creating a synchronous shape change of the crystalline lens.3,6 The CM is the dynamic driver or agonist of the accommodative apparatus. Uniquely, the 3 fiber directions—circular, meridional, and longitudinal muscles—pull the crystalline lens forward and inward to create the centripetal forces that produce accommodation during phase 1, which is the phase from disaccommodation to accommodation.7
Lens: Due to its biconvex shape, the lens provides changing optical power for phase 1 and 2 to adjust for variable distances. Through forces supplied by the BMCC and CMs, the zonules relax, releasing tension from the lens capsule to shift from phase 2 to phase 1; moving from disaccommodation to accommodation. The lens changes into its natural rounded shape to focus on near distances. Similarly, when the lens shifts from phase 1 to phase 2, moving from accommodation to disaccommodation, the zonules are pulled taut by the elastic recoil forces of the BMCC to pull the lens into a flattened shape.8,9
Bruch’s Membrane-Choroid Complex: The BMCC consists of multiple layers, including the basement membrane of the retinal pigment epithelium and the choriocapillaris basement membrane. This elastic structure extends around the eye and is essential in the accommodation/disaccommodation cycle. Due to its connections to the ciliary body, the BMCC pulls the lens into a disaccommodation position and is the primary dynamic force to achieve disaccommodation.8 During accommodation, the BMCC serves as a way to store potential energy for disaccommodation. The BMCC is inherently elastic and pulls backward on the lens, storing energy like a spring 8,9 (Figure 2).
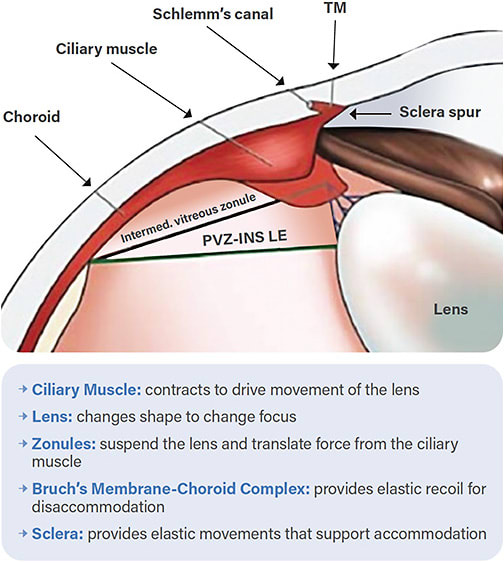
Hooke's law states that a spring extends proportionally to the force applied. Applying Hooke’s law to the BMCC during accommodation, we can see that the elastic stretch of the BMCC stores energy proportionally to the magnitude of the force with which the CM pulls against it.8,10 Upon release, the BMCC acts like a slingshot to drive disaccommodation (Figure 3).
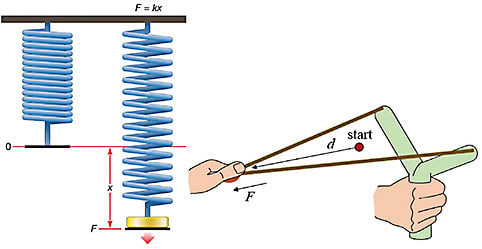
Zonules: A plurality of zonular pathways connect to various components of accommodative anatomical structures. The zonules anchor into the pars plicata and the BMCC. Zonules serve as bidirectional spring forces during all phases to transfer forces through the capsule to the lens (Figure 4). The zonules, which suspend the lens, slacken upon accommodation, allowing the lens to ‘round up’ into its inherent position.11
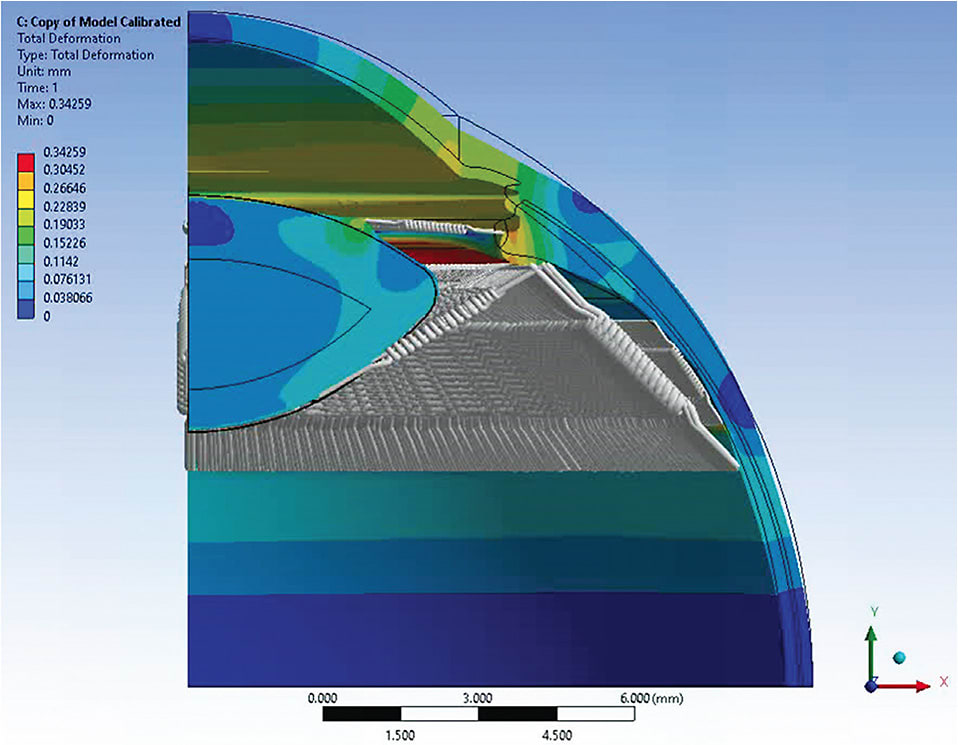
Sclera: The sclera connects to other components of the accommodative apparatus. Like the BMCC, the sclera is viscoelastic, and during ciliary contraction, it bows inward,12 while the cornea makes compensatory movements outward (Figure 5).
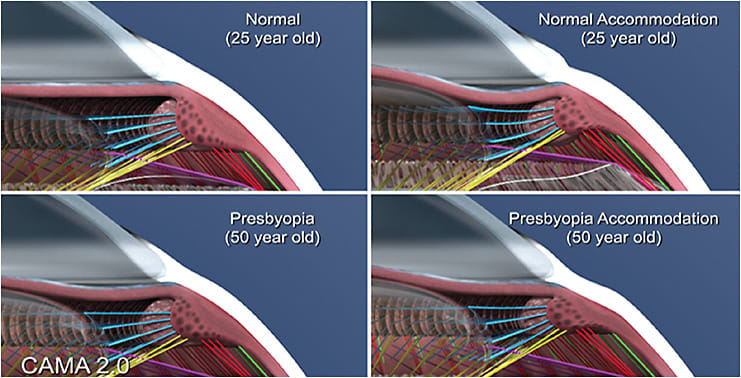

Kinematic Chain of Accommodation
A Kinematic Chain is a way to describe a sequential set of motions that occur through connected anatomical structures that jointly translate the resultant effective forces that produce specific dynamic movements in a rigid body.14 Typically, this analysis applies to rigid bodies, but we also extend its use to soft tissues. A complicated Kinematic Chain within the eye responsible for DRoF includes a preload phase 0: prestretch (maximally disaccommodated); phase 1: disaccommodation → accommodation chain reaction; and phase 2: accommodation → disaccommodation chain reaction. Understanding the Kinematic Chain can illuminate the normal biomechanical functions that provide efficient DRoF, as well as pinpoint specific causes and sources of the biomechanical dysfunctions that produce ineffective DRoF, such as those that occur with progressive age, such as presbyopia. The phases of DRoF help to illustrate the chain reactions that impact DRoF function. In the next and final article in this series, we will discuss the biomechanical dysfunctions in the Kinematic Chain to identify key anatomical structures contributing to the loss of focusing power that occurs with presbyopia.
Conclusion
DRoF is a neuromuscular function involving explicit biomechanical interactions between anatomy with highly specialized roles in the Kinematic Chain. There is a chain reaction at play during DRoF that is bidirectional and highly dependent upon the mechanical laws of physics under which all muscular contractions in the body operate. In addition to the key anatomy we have outlined here, there are even more layers of biomechanical complexity, including the Stiles-Crawford phenomenon of the appearance of light as it enters the center of the pupil,15 and retinal movement with respect to the pigment epithelium due to hinges in the cilia.16 The presbyopia puzzle exists because we have failed to understand these fundamental structures' critical dynamic roles. As we analyze the sequences in the Kinematic Chain, we can unlock the mechanisms responsible for presbyopia's pathogenesis which opens the door to treating the real problem instead of this age-old conundrum. ■
References
- Hipsley A, Bocskai Z, Price E. Dynamic range of focus of the eye: understanding biomechanics and kinematics: part I. Presbyopia Physician. 2023;3(1):25-28,43.
- Kasthurirangan S, Glasser A. Characteristics of pupil responses during far-to-near and near-to-far accommodation. Ophthalmic Physiol Opt. 2005;25(4):328-339.
- Padulo J, Laffaye G, Ardigò LP, Chamari K. Concentric and eccentric: muscle contraction or exercise? J Hum Kinet. 2013;37:5-6.
- Reed J, Bowen JD. Principles of sports rehabilitation. In: Seidenberg PH, Beutler AI, eds. The Sports Medicine Resource Manual. W.B. Saunders; 2008:431-436.
- Kaufman PL, Lütjen Drecoll E, Croft MA. Presbyopia and glaucoma: two diseases, one pathophysiology? The 2017 Friedenwald Lecture. Invest Ophthalmol Vis Sci. 2019;60(5):1801-1812.
- Knaus KR, Hipsley A, Blemker SS. The action of ciliary muscle contraction on accommodation of the lens explored with a 3D model. Biomech Model Mechanobiol. 2021;20(3):879-894.
- Prosak TP. Forward and Inward Movement of the Ciliary Muscle Apex with Accommodation in Adults. Thesis. The Ohio State University; 2014. Accessed June 27, 2023. https://etd.ohiolink.edu/apexprod/rws_etd/send_file/send?accession=osu1397425606
- Strenk LM, Guo S, Lu K, Werner L, Strenk SA. Force of lifelong crystalline lens growth: chronic traumatic mechanical insult to the choroid. J Cataract Refract Surg. 2022;48(3):342-348.
- Ugarte M, Hussain AA, Marshall J. An experimental study of the elastic properties of the human Bruch’s membrane-choroid complex: relevance to ageing. Br J Ophthalmol. 2006;90(5):621-626.
- Hooke R. De potentia restitutiva, or of spring explaining the power of springing bodies, vol. 1678. London, UK: John Martyn.
- von Helmholtz H. Helmholtz’s Treatise on Physiological Optics. (Southall JPC, ed.). The Optical Society of America; 1924.
- Croft MA, Nork TM, McDonald JP, Katz A, Lütjen-Drecoll E, Kaufman PL. Accommodative movements of the vitreous membrane, choroid, and sclera in young and presbyopic human and nonhuman primate eyes. Invest Ophthalmol Vis Sci. 2013;54(7):5049-5058.
- Goldberg DB. Biomechanics of the lens and hydrodynamics of accommodation. In: Pallikaris I, Tsilimbaris MK, Dastiridou AI, eds. Ocular Rigidity, Biomechanics and Hydrodynamics of the Eye. Springer International Publishing; 2021:117-126.
- Chain Reaction: Successful Strategies for Closed Chain Testing and Rehabilitation. Wynn Marketing, Inc.; 1992.
- Singh N, Atchison DA, Kasthurirangan S, Guo H. Influences of accommodation and myopia on the foveal Stiles–Crawford effect. J Mod Opt. 2009;56(20):2217-2230.
- Burnside B, Nagle B. Chapter 3 Retinomotor movements of photoreceptors and retinal pigment epithelium: mechanisms and regulation. Prog Retin Eye Res. 1983;2:67-109.